Article by Richard Heidmann, Association Planète Mars vice president – English translation by Etienne Martinache.
After having analyzed the targets assigned by SpaceX to its project of an Earth-Mars transportation system which is supposed to set up and serve a Martian settlement (see “l’étude MCT” on the site www.planete-mars.com), we decided to address the issue of an essential aspect of the feasibility of the project, the design of the living areas (pressurized enclosures).
This aspect was subject to many proposals, even though most of previous documented studies applied to upstream phases of human presence, those of exploration from a temporary base or from a permanent base with few residents and limited self-sufficiency. The consequences of the specific constraints related to a mass production of these enclosures, essentially from local resources, have seldom been considered.
1 Framework scenario of the study
We have made assumptions in line with the expected operational performances of the MCT and with the results of an economical model of the settlement, considering that its main purpose would be to provide the settlers with a home and associated services.
This model, still in its preparation stage, will not be detailed here. Its purpose is to estimate the required size of the settlement and a price list in order to meet a financial balance in steady state, taking into account:
- Costs (transportation, purchase of supplies, maintenance, salaries, etc.);
- Duration of the residents’ stay;
- Lifespan of the settlement.
Customer residents (paying residents) would belong to two categories: scientists assigned to research and technology projects by scientific institutes or private companies, and (rich) tourists willing to spend a part of their wealth on their life’s dream: living on another world. The other residents will be employees, or independent contractors, who, also attracted by this great adventure, will enlist to carry out the numerous duties required to ensure the proper operation of the settlement. Their professional skills will meet high standards of qualification. Of course, employees’ costs (transportation, stay) will be paid and employees’ incomes will be attractive. All residents’ selection criteria will also include rigorous psychological screening.
2 Essential data
Considering the expected capacity of the MCT and the preliminary results of the economical model, here are the minimal values and constraints which we have taken into account:
- Payload of a MCT vessel: 100T, including a maximum number of 100 passengers (who make up 50T including their food and water supplies).
- Size of the settlement: 1000 residents (the limiting factor is the number of flights per synodical period).
- 425 paying residents (including 300 tourists).
- Lifespan of the settlement: around 20 years.
- Habitat private area: 30 m² per resident.
- Cultivated area for food production: 60 to 90 m² per resident.
- Number of outward flights per synodical cycle: 6.
- Traffic per synodical cycle: 570 passengers in each direction (outward and return) in steady state, and 280T cargo on outward flights. This high level of passengers’ traffic comes from the assumption that most of the tourists will stay on Mars during one cycle only (18 months) and most of the employees and contractors will stay one or two cycles (44 months). This scenario is obviously not economically optimized, since it increases the ratio of the travel costs in the cost balance, all the while requiring an increase of the size of the MCTs fleet.
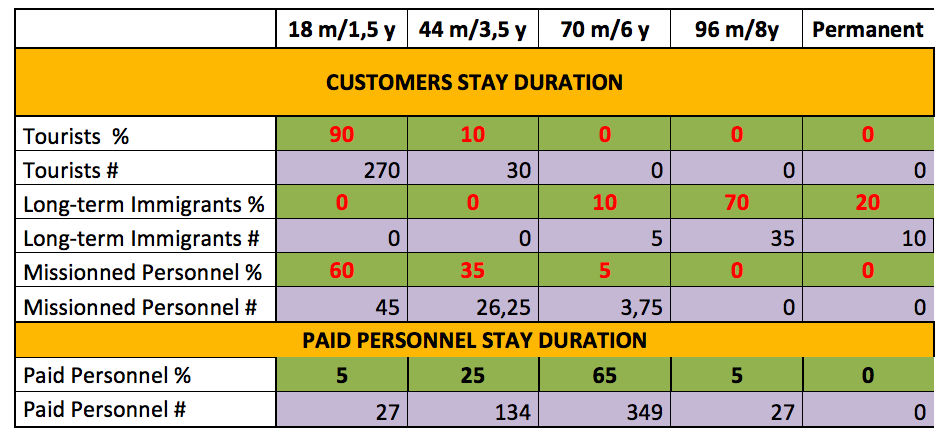
Working assumptions of planetary stay durations, for various residents’ categories.
3 General considerations
3.1 Constraints of this initiative
A 1,000 residents settlement may seem not really challenging if you consider the perspective of a future advanced planetary civilization. But when we look at the project in some detail, we soon realize the numerous obstacles it will face, even at this stage. Those mainly result from the transportation cost (even with reusable MCTs), the low launch rate of transportation vessels, the large logistics required to provide the base with energy and food supplies, and the constraints of Martian environment. Last but not least: the amount of initial investment, which will restrict the design of the habitats and impose the setting up of in situ production means.
3.2 A decisive orientation of the resident concept
Considering a model of Martian settlement dedicated to provide accomodation and services to customers, whether tourists or professionnals, designed as a long term business, constitutes a radical conceptual reorientation compared to conventional scenarios, which generally just consider the perspective of developing scientific exploration. One may object that this position is unrealistic since the actual feasibility of this concept, whether technical, financial or human, has not been demonstrated yet. But this idea is fertile enough and, in a certain way, already in sight, so that it is worth looking deeper into the issue. As said before, this reorientation brings up new economical imperatives, but also implies modifying the way to envision the living conditions of the residents: in this regard, the Martian population will not fit anymore with the conventional models of astronauts on orders, ready to numerous sacrifices provided that their safety and scientific productivity always come first, or of settlers who made a radical choice: an irrevocable exile to another world.
3.3 Two outstanding consequences of the MCT hypothesis
Lacking further information, let us keep our initial assumption of a 100 tonnes payload, including a maximum of 100 passengers; taking into account the results of our economic model we can then point out two important aspects of this scenario.
First, even considering a very low launch cost (200 M€, which is nevertheless higher than the target cost ambitioned by SpaceX), we find out that the amount of wages is lower than half the transportation costs, despite the long duration of the missions and considering the high wages (9,000 to 40,000€/month) required to represent a real incentive for the volunteers to go for it. This strengthens the feasibility.
Besides, the necessity to achieve a minimum number of flights (mixed) per synodical period in order to ensure sufficient passengers traffic (6 in our model) leads to a rather oversize cargo capacity which makes it possible to adhere less strictly to the principle of maximal use of local resources (cf. § 4.3). This leads us to consider that a certain quantity of goods, whether indispensable or prized, but the in situ production of which is unrealistic or prohibitive as far as investment is concerned, will be imported from Earth, provided their unit weight remains reasonably low. When looking at the needs of a settlement, two categories of goods in particular may be considered:
- Food supplies: complete self-sufficiency would be hard to achieve, since it would imply vast cultivated areas (from 60 to 90 m² per resident), numerous greenhouses, it would also be hazardous for the inhabitants’ health, and morale as well; why should they be deprived of calorific, popular and nutrients rich dietary supplements? Such as coffee, chocolate, (small) fruits, or various kinds of candies typical of many culinary cultures;
- Frame parts: in order to produce building materials, we shall need some specific chemical additives (for instance Na2O, K2O for glass), it is of course possible to get them in situ but importing them from Earth would be more cost effective, as long as their quantity remains reasonably low. We should even consider importing some light structure parts, too complex to produce, such as for instance frames and linings in carbon composite.
4 Some principles of the habitats design
Living spaces, meaning pressurized enclosures, may be divided up into four categories, each featuring specific characteristics: private areas (rooms, studios, suites …); public areas (restaurants, sports halls, showrooms, services, shops); research and development laboratories (public and private); « industrial » areas.
We shall limit ourselves to the first two categories, which explicitly feature two main obstacles, to wit on one hand mass production (condos and parts of public areas), on the other hand the necessity to avoid dull surroundings such as those of barracks or “technical” environments. A real challenge, considering the project’s constraints.
4.1 Burying ourselves as least as possible
Burying habitats is often recommended in order to cope with the risk of ionizing radiations overdose. For instance we may consider using a tunneling machine, the size of which would remain compatible with the cargo capacity of the MCT (5m diameter, maximum speed: 1m per sol, power: 150 to 200kW and weight lower than 100T), to dig tunnels for cave dwellers; but this means that only the condos close to the tunnel’s entrance would look out on the outside. An attractive way to implement this burying concept is to combine it with the art of masonry, as shown by Bruce McKenzie’s detailed studies (Homestead Project). He advises to dig wide trenches able to accomodate brick vaults and cover them with regolith. Trenches are fitted with skylights in order to avoid exclusively artificial lighting. Here and there, in some specific places exposed to a limited amount of radiations, some windows make it possible to catch a glimpse of the outside.
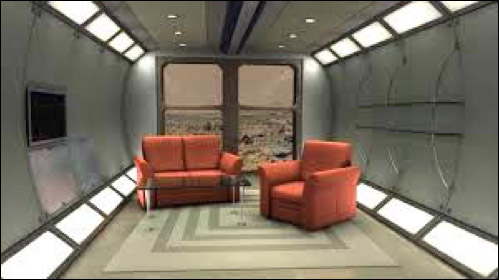
(DR)
This option features a characteristic which hardly makes it desirable for private areas. Brick masonry, not easy to implement in the harsh Martian environment – it is indeed difficult to conceive of spacesuited bricklayers climbing walls and walking on scaffoldings – does not seem realistic considering the necessity to mass produce living structures to cope with the expected high growth rate of the settlement. But above all, in this scenario of offer of Martian residence, it would definitely be inconsistent to doom to a moles’ life such people, whether rich tourists, employees or contractors dedicated to operating the settlement for whom this wonderful stay on Mars will represent a lifetime’s dream; these enthusiasts will definitely want to feel that they are actually on Mars. Moreover, easy access to the wonderful Martian landscape will undoubtedly constitute an invaluable psychological support for all residents. However, this option is conceivable for public areas (see section 6).
Actually, rather than burying the buildings, the radiation issue could be tackled more effectively by applying a design principle which may be called the « cap effect ». This principle relies on two facts:
- regarding exposure control: during the mission, the main contribution to the global dose will remain, however hard we try to minimize it, the dose absorbed during the interplanetary transfer phases; as an order of magnitude, for a stay lasting one cycle (18 months spent on Mars), the dose absorbed at ground level will be around a few cSv (with protective habitats and solar storm shelters (anti-SPE [1] shelters) while it will reach 50 cSv during the two transfers. Therefore, trying to bring the dose to zero during the planetary phase would not be a rational objective, all the more so that it would also mean giving up on all surface trips; actually, as far as his health is concerned, the Martian traveller will be supposed to manage his personal dose by continuously adjusting his exposure level, in order to come back to Earth with a deliberately accepted total dose (and relevant health risk)[2];
- regarding the origin of ionizing radiations: cosmic rays (Galactic Cosmic Rays, GCR) originate from all directions of the sky; but in a habitat, the resulting dose will be reduced pro-quota of the part of the celestial sphere which is actually visible from the relevant location. On the Martian surface, the faraway skyline naturally blocks off half the amount of radiations; if the settlement is built at the bottom of a hill or a cliff, a further reduction will apply; finally, making good use of the « cap effect », we can design a building featuring a continuous gradation of sky views, which makes it possible to keep the most naturally crowded places (such as beds, offices, restaurant tables …) in blind areas, while providing halls and gangways (or even places designed to gaze at the landscape) more or less open to outside view and let ample external light flow inside. For instance, to see what it may look like, let us consider a 20m diameter community geode (orange ground) topped by a 8m inner diameter « cap ». This « cap » in duricrete could feature a hanging garden and accomodate completely protected areas (such as offices, laboratories).
[1] SPE (Solar Particles Events), powerful and erratic puffs of protons, are not a real danger since their energy range is much lower than GCR energy range, thus make it easier to cope with (a layer of water or polyethylene 30 to 50cm deep are enough to stop them).
[2] For the record, the present rules (applicable to the ISS) state that the maximum dose absorbed by a human being must not exceed 100 to 400cSv over his whole lifetime (depending on age and sex), this dose corresponds to a 3% increase of death risk by cancer.
4.2 « Unspatializing », customizing and keeping social friendly surroundings
The residents should also benefit from living conditions as less « spatial » as possible and, in order to lure potential tourists, as attractive as possible. While this is obviously an essential aspect, we shall not elaborate further because this is the work of specialists, such as architects and psychologists. We shall just mention it as far as pressurized enclosures are concerned, we shall not address the issues of layout of living areas (in the broad sense of the term); let us just point out that the design of the condos must be flexible, according to the visitors’ likings. This means that it should be possible to customize their interior design, furniture and even, in a certain way, their internal arrangement. As for the overall design, it will be difficult to avoid providing pathways in the form of hotel corridors: no « villas » will be built, or only as an exception, since it is highly desirable to move easily from any place of the settlement to any other place without the necessity to don a spacesuit.
Public areas will play a crucial role but cause specific problems, because of their sheer size. The assumed growth rate of the settlement, 100 residents per synodical cycle, means that each 26 months a new restaurant and relevant social area should be added, able to serve one hundred guests (in two shifts). One or two other modules should be built upon each cycle too (see § 6.1). Scientists and contractors will work either in modules derived from standard private habitats, or in areas located in the « caps », or in customized industrial modules or laboratories (excluded from the scope of the present study).
4.3 Using local resources as much as possible
The weights of construction materials required to build enough habitats to accomodate 100 additional residents each 26 months (that is one per week), combined to the high cost of interplanetary transfers, is a strong incentive to maximize the use of local resources. Indeed, one of the major advantages of Mars is precisely its capacity to provide relatively easy access to most of necessary resources.
One critical aspect remains, however: energy (on this matter see § 7.7 and 7.8). Energy production is a challenge: oversize heatsinks for nuclear reactors[3], or huge fields of solar panels for photovoltaic generators[4]. Now, a large amount of power will be required at an early stage of the settlement installation, mainly to produce construction materials. In the long run, local mass production of solar panels may possibly meet the needs of the settlement, but in the short run the settlers will definitely need small electronuclear generators, buried in the regolith (typical power, weight: 5 MWe, 10tons).
Another important resource, nitrogen, will be especially difficult to retrieve. This gas is present in the Martian air, true, but its concentration rate in volume[5] reaches only 2.7 % of a very thin atmosphere (6 to 7hPa, compared to 1013hPa on Earth). Nitrates, another possible source of nitrogen, have been spotted in the Martian ground, but also at a low concentration. Now, the pressurized enclosures are supposed to feature a pressure of around 500hPa, including 50% nitrogen, which makes up for a considerable amount of nitrogen, even for the first batch of 100 residents only. Extracting 1kg nitrogen from the atmosphere, which roughly corresponds to the needs of 3.2m3 of habitat volume, requires processing 1,700m3 Martian air and 10kWh energy (Utilizing Martian Resources for Life Support, C. MCKay). Considering one batch (6m, 560m3) each two weeks, you need to process 870m3 air per hour, meaning around 240l/s, the power required by the whole process reaches circa 5kW. These orders of magnitude show that it will be possible to obtain this scarce resource, although if not easily.
It is obvious that the low level of availability of these two resources will bring some restrictions to the design of the habitats.
The following table is a recap of the raw materials available to build the habitats.
The diagram below details the lines of processes leading from the sources (cones) to the materials and products (cylinders) considered as a priority in the present study.
[3] On Mars, no rivers or seasides are available to drain the calories. Moreover, the heat transfer technology necessary for the settlement to use this power is very complex (coolant, heat transfer fluid, facilities…).
[4] Considering various unfavorable factors, the average power yielded over one year by fixed solar panels (to which batteries must be connected) is only 60W/m².
[5] It is also possible to extract argon from the Martian atmosphere (its present concentration level is 1.6%) in order to use it as a buffer gas.

In Situ Resources Utilization for Colony Building
As already seen in section § 3.3, some exceptions should be brought to the principle of resources self-sufficiency, which in a certain way is allowed by the assumptions regarding the performances of the MCT and the Earth-Mars traffic. Except food supplies, which will not be discussed here, we should in particular consider importing the following resources from Earth:
- Electronuclear generators: their power will be limited by the area of relevant heatsinks and by the temperature around the turbines of the turboalternators; let us assume a 5MWe unit power (see « Essai d’analyse technique d’une colonie martienne » on APM site); they will be indispensable at the beginning of the settlement building phase, before a solar panels in-situ production unit is operational;
- Parts of structures: those needing technologies which it would not be profitable to set up early on Mars, in particular those requiring high dimensional precision or high quality surface finish, provided their weight remains reasonably low;
- Various habitat appliances: heating, ventilation, toilets, furniture (although a part thereof may be manufactured from local resources: plastics, plant fibers);
- Plexiglas sheets: considering the almost complete lack of oxygen in the Martian atmosphere, therefore absence of any ozone layer, the UV radiation rate on the surface is extremely high (six hundred times the UV rate on Earth’s surface). Fortunately, Plexiglas, a light and cheap material, easy to machine, can be used to block out UVs effectively. Plexiglas could be produced in-situ from available resources, but its production process is complex, four steps are necessary which require specific facilities. Importing it from Earth will be easier.
4.4 Optimizing production and assembly means
It is necessary to limit the material and financial investments during the upstream phase of the settlement construction, and reduce as much as possible the time necessary to set up the facilities on site (determined by the maximum possible cargo traffic allowed by the MCT system).
However in our scenario, mass producing « condos » will benefit from the use of glass and steel, these two sectors require high temperature enclosures (1550°C for glass, 1200°C for steel). Glass prevents visual confinement and steel makes it possible to obtain solid frameworks, thus contribute to the structural safety of the habitats and make up an adequate interface with the windowpanes. Plastics, even the most reliable of them, are not mechanically strong enough for pressurized enclosures (insufficient bending strain and creep resistances); most of them are flammable, thus constitute potential safety problems.
As for private accommodation areas, we advise modular concepts based on moderate size and mass units (size: 6m x 6m x 6m, mass:7 to 8 tons per hexagonal cell), which enable:
- Time savings and working mandays reduction due to a mass production effect;
- Designing production means small enough to be dispatched on Mars: pre-connected « production lines » ready for use once landed on the Martian surface, already installed in built-in frames set up in inflatable workshops;
- Easy handling of the modules, thus enabling easy assembly, transportation and installation of finished products on site, operable by a small number of operators and not requiring hoisting means or levelling works of such size and power as not to be compatible with work in Martian environment.
Those are the principles which led us to the concept of private accomodation areas shown in section 5 hereafter.
As for public areas, which are an integral part of the settlement concept such as considered in this study, the range of potential technologies can, and must, be expanded. The most attractive concept is definitely the transparent dome (combined with the « cap » effect), but the problems of its implementation rapidly increase as a function of its diameter. But we shall see that (section 6), since those areas are not subject to the same constraints as the condos, alternative solutions may possibly apply initially.
5 One example of habitat concept
5.1 General configuration
This concept consists in assembling elementary modules, each one a six-sided cylinder (hexagon shaped), each cylinder is 6m long and each one of its hexagon sides is 3m wide. The flat surfaces of these modules are made up of four tempered glass flat panes, each one 3m long, 1.5m wide and 30mm thick. A thin additional Plexiglas layer provides for UV filtering. The tempered glass, which is at least five times stronger than ordinary glass (due to surface prestressing effect resulting from the tempering process), is double layered, which makes it possible to eliminate the risk of material damage (caused by an impact)[6]. These panes are mounted on a steel frame made up of six longitudinal ridges (in red below) in order to transfer the pressure strain onto the ends of the overall structure (the stress value is close to 1,200kN per hexagon) and onto the 3m longitudinal and transversal angle bars. Some of these angle bars support the rabbets, which are interfaces between the glass panes and the structure designed to transfer the pressure strain and ensure proper airtightness, all the while allowing motions caused by natural distortion and expansion of the structure. Finally, the hexagonal shaped ends of these modules are prefitted in order to facilitate their assembly while keeping proper required airtightness.
Living areas will be made up of several individual units, each one including four such modules, arranged as shown below, these modules will be assigned to: living area proper (1 cell, blue), gangway (1 cell, orange) and greenhouses for food production (2 cells, green).
Each condo would in principle be fitted with a partition wall (blue cell level) designed to separate it from the neighbouring condo. These walls are removable, thus making it possible to increase the length of the « condos ». Each living cell proper, 6m high and 30m² flat area, is divided up in three levels:
- A « cellar » accomodating technical services, various hydraulic and electric fluids circuits, which is also a storing area for the equipment of their inhabitants, including their Mars suits;
- A main living floor, including shower and toilets;
- A mezzanine located « benath the attic ».
This basic configuration features a 60m² living area and a 60m² greenhouse flat area, which is probably enough to provide a total amount of 120 to 150m² cultivated area. This corresponds to the minimum needs of two persons, considering the assumption that part of the food supply (10%?) will be imported from Earth. A dozen such units will constitute a line of buildings connected by a common gangway, this gangway leads to a connecting door giving access to the rest of the settlement. The purpose of this door, normally closed, is to limit the consequences of a possible air pressure drop occurring anywhere in the settlement. It must be wide enough to bring in bulky equipment. On the other side of the line of buildings, an airlock gives direct access to the outside.
All cells of the whole set of buildings are isobaric (0.5 bar) therefore it is not necessary to install glass panes between the cells; but the structure frame is still required, it should even be strengthened because of this absence of panes. However it is preferable, for sanitary reasons, to keep the line of greenhouses isolated from the line of habitats. The chosen arrangement provides the settlers with an enjoyable point of view, away from the infrastructure (greenhouses and gangway), both on the surrounding landscape and on the vegetable patches.
All cells of the whole set of buildings are isobaric (0.5 bar) therefore it is not necessary to install glass panes between the cells; but the structure frame is still required, it should even be strengthened because of this absence of panes. However it is preferable, for sanitary reasons, to keep the line of greenhouses isolated from the line of habitats. The chosen arrangement provides the settlers with an enjoyable point of view, away from the infrastructure (greenhouses and gangway), both on the surrounding landscape and on the vegetable patches.
[6] By the way, an argument often used to justify burying the settlement is the hazard of micrometeorites to such pressurized enclosures. This is not an issue because the Martian atmosphere pressure at ground level corresponds to the air pressure on Earth at 35 to 40km altitude, which is considerably lower than the altitude where micrometeorites disintegrate and turn into shooting stars on Earth (around 80km). Larger objects only must be considered in the risk assessment, but they are also much more seldom.
5.2 Efficient protection against radiations
To provide the settlers with an efficient protection against radiations, without turning them into moles, we suggest to use water and resort to the « cap effect » mentioned above. Water is one of the most efficient protective materials, less than liquid hydrogen but equivalent to polyethylene (CH2n) (see NASA graph hereafter); the habitat “roof” sections and backside are covered with (fixed) Plexiglas panels containing a layer of water ice 50cm thick, which practically divides by five the dose coming from relevant directions. The south face of the habitat module is fitted with similar panels, they are removable, thus (for this reason) thinner (30cm thick, dose divided by four). As for greenhouses, experts’ opinions vary regarding the capacity of cultures to withstand a massive Solar Particle Event (SPE) (though it would be easy to obtain the necessary data with laboratory experiments …); let us remain on the optimistic side, but if necessary it would be possible to fit the south faces of the greenhouses modules with removable panels too. As for the habitat, beyond its role of anti-SPE shelter, the removable front panel gives the residents the choice between: either looking for maximum protection without any possibility to see outside (only daylight will cross the ice); or enjoying the landscape (through the glass panes) and using « the cap effect » to minimize the dose (see picture below).
On Mars, without any protection, Curiosity measured doses comparable to those received by astronauts in the ISS (where some of them already spent one year). We may therefore consider that this protection policy will deal efficiently with the radiations problem for the time spent in the habitats.
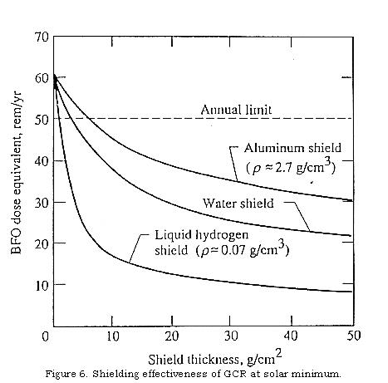
Residual dose (in rem or cSv per year) received at the bone marrow level (Blood Forming Organs) in interplanetary space for various materials, as a function of their thickness (in g/cm²). (doc. NASA)
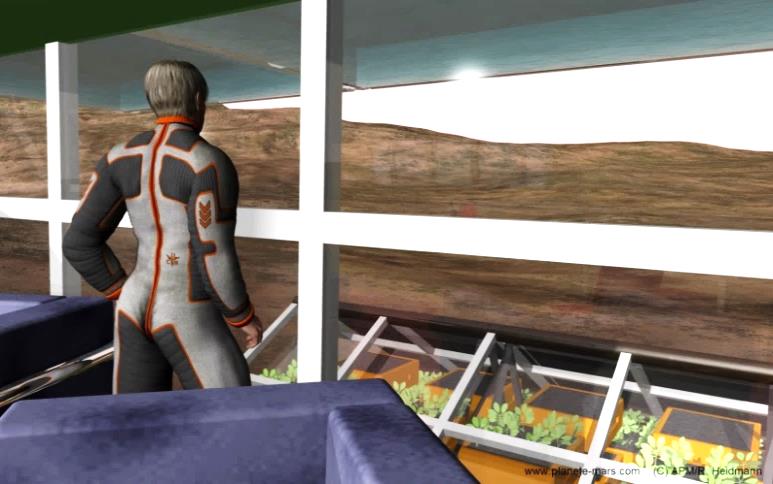
It is possible to adjust the dose of ionizing radiations by masking the heavenly vault with mobile water ice panels. Hereabove, they have been moved upwards in horizontal position, the resident thus benefits from the « cap » effect.
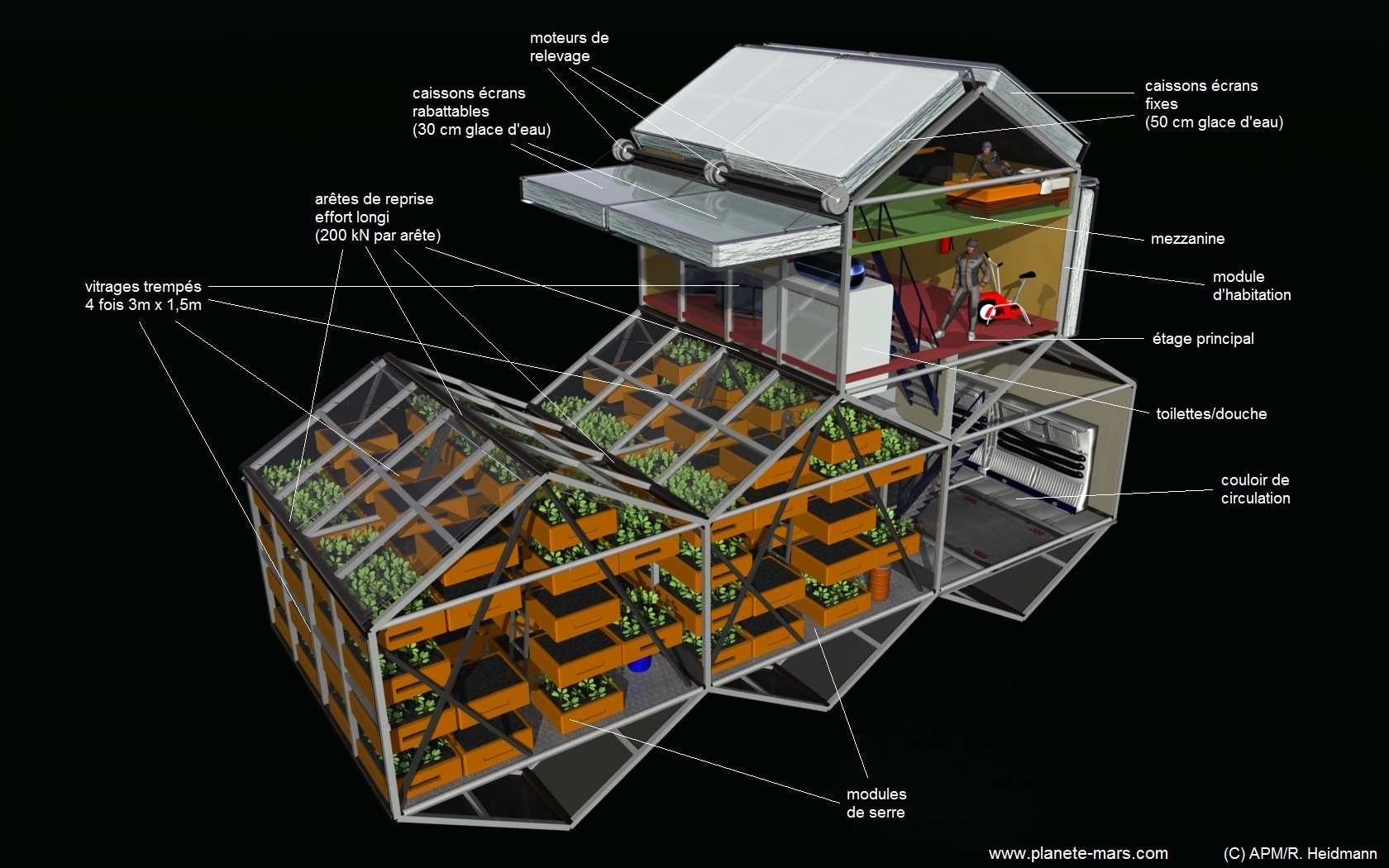
Living unit, with two greenhouse modules, for two residents. Cultures are shown here in casings, but we could as well go for hydroponic cultivation (not discussed here). Note: the frames shown here are steel; the greenhouses shown here are supposed not to need any anti-SPE protection walls (removable)
It will be necessary to send on Mars the whole mounting and installation facility of such panels, spatialized, ready for use and built in the frames of connectable workshop-modules. We tried to estimate the amount of work implied (see section 7); this is a critical part of the feasibility of the project. The production rate is of course not to comparable to terrestrial industrial standards, but if we need one 6m module each two weeks, this means 18 x 4 = 72 panels, that is 5 panels per day.
5.3 Some sizing data
Note: Should 3T steel per week turn out to exceed the production capacity of the settlement, the ridges and angle bars could be imported from Earth, and could then be made of carbon composites, much lighter than steel.
6 Designing community areas
6.1 Importance of community areas
We can easily list the various types of areas:
- Restaurants and social areas, including bar and kitchens, able to accommodate one hundred guests (in two shifts);
- Fitness centers, which may be provided with various types of exercise gear, bodybuilding and stretching gear, including a swimming pool (fitted with a Martian gravity diving board!);
- Shopping areas, relaxation lounges, purchase or exchange of goods for everyday life;
- Cultural areas, for shows, conferences, meetings;
- Training centers and schools, for adults and children (provided children are allowed in this first settlement…);
- Medical area, hospital, emergency services and administration offices.
These areas should be wide enough to accommodate simultaneously at least 100 or 200 residents. Their user-friendliness and the quality standard of their specific features will definitely determine the quality of life in the settlement, therefore its success as an exceptional residence.
As for the pace of the building program, one additional area of the first category will be required upon each synodical period, in order to put up and feed one hundred additional residents. However, the pace will be lower for the other areas. It is reasonable to consider adding two new areas per synodical period (that is each 26 months).
6.2 A problem different from the habitats problem
The design of these pressurized areas features at least two advantages which, fortunately, make it possible to ease off a bit the technical constraints and widen the range of possible solutions.
First, although their unit volume is substantial, we do not face anymore the quasi industrial pace of building of habitat modules (one additional resident per week). We might thus consider applying technical solutions requiring more time than habitat modules.
Besides, although a transparent dome is clearly an ideal structure for a Mars settlement, since it will make the residents feel actually present on the planet’s surface, simpler solutions, easier to implement, even if they do not allow any possibility to see outside, should be accepted by the visitors of the first « shifts ». They should understand that being a pioneer implies some sacrifices.
6.3 Starting option
If we assume that the first buildings may be designed so that its residents will be almost cut off from the outside, then two construction techniques may be considered:
- Building vaulted areas with bricks, this option, described in section 4.1, was not adopted for the habitat modules. Its architecture and low energy needs are attractive. On the other side, it implies a lot of earth moving. But unlike individual habitats, the necessary works may be spread over a long time, thus will not require excessive means. Producing bricks from clay is easy, it just requires a low temperature oven (400°C); duricrete also makes it possible to produce prefabricated modules, by casting or forming, thus reducing the time spent on the construction site. However, building up the vault brick by brick will require a bricklayer robot, since it is not reasonable to consider workers doing such a long job in spacesuits. The Homestead project (Bruce McKenzie) opted for this solution and proposed quite enjoyable areas. But even with skylights and a few windows here and there, residents will basically be cut off from the outside.
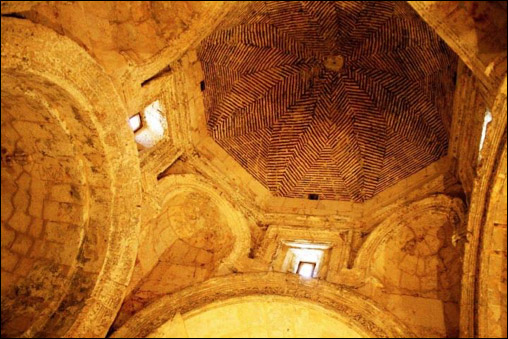
This may look like the interior of a pressurized Martian enclosure, designed as per the ancient technique of vault building. (DR)
- Building by 3D printing (“3D building”): rapid progress of this technology has made it an attractive option for planetary habitats, it has even been tested already; ESA obtained convincing results in this field (in a lunar context) and recently, NASA launched a contest (this time for Martian environment). The winning project made use of water ice as raw material! Various processes have been devised to implement 3D printing in planetary habitat construction. The specialized company Fabulous[7], for instance, proposed NASA to set up an automated warehouse using iron, which is abundant as iron oxyde in the Martian soil, to build the structure of a double walled dome fitted with a built in water shield. The ESA project resorted to additives which facilitate regolith printing. Let us point out that in both cases, essential functional parts are imported from Earth, whether an airtight envelope, or acting as a template, or an airlock leading to the outside. Provided the size of the dome remains reasonably low (15 m?) this is a promising technique indeed.[7] Advised by the “Association Planète Mars”
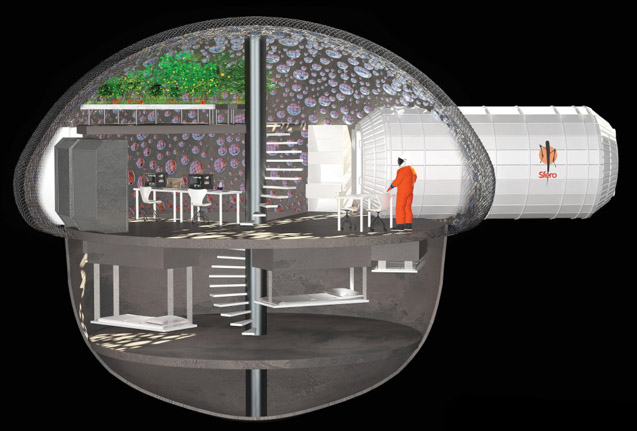
The Sfero project proposed to NASA by Fabulous, advised by APM. (doc. Fabulous)
6.4 How to make dome covered areas?
Even if, as described above, the first community areas could be « buried », the architects of the settlement will definitely want early on to build enclosures having the largest possible view of the outside, though also, as explained in section 4.1, featuring areas with various levels of exposure to ionizing radiation. But for this type of areas, much larger than individual condos, huge flat windowpanes are ruled out. This is due to the fact, not easy to grasp intuitively, that the pressure difference between the inside and the outside of the enclosure (500mbar compared to 6 or 7mbar) applies a considerable force to the panes (5 tons/m²). This is why many very appealing concepts of Martian habitats will unfortunately never see the light of day. To be frank, the habitat design concept shown in the present study makes use of flat panes indeed! But we made it sure that their size (3m x 1.5m) is small enough that their resistance to pressure is sufficient, provided we go to 3cm thick tempered glass and add strengthening angle bars (see figure 5.1). The fact remains that this architecture cannot be generalized to community enclosures, the same comment applies to the conventional shapes (parallelepipoids) of our buildings on Earth.
To break the deadlock, the (transparent) dome concept looks like an appropriate solution, both more rational on a mechanical resistance point of view (the sphere is the lightest shape able to contain a given pressurized volume) and more user friendly as for the number and variety of arrangements and leisure facilities allowed. Unfortunately, a certain number of problems arise, considerably increasing with the size of the dome (diameter). So:
- it is often said in the literature that the dome could be a simple « balloon », made up of a flexible membrane kept in shape by the 0.5 bar inner pressure of the dome, this balloon could be half buried or even just moored to a cradle lying on the surface; but can we really design an envelope large enough, airtight, able to withstand the pressure and block out UV light? It will likely need to be imported from Earth, and it does not look reasonable, given the huge mass involved, to consider domes more than 10m wide;
- half burying the dome implies a colossal amount of earth moving, since the amount of work grows as the cube of the diameter;
- an armatureless envelope would collapse in case it tears off;
- if we decide, for radiation protection purpose, to cover the pressurized envelope with ice or regolith, this collapse would be sudden and dramatic;
- it has also been said that the weight of such a cover would withstand the pressure applied to the envelope, but this is just true on the top of the dome, and almost not at all on its sides;
- it is often recommended to use plastics to manufacture the envelope, because several kinds of plastics could be produced on Mars; but its mechanical characteristics are poor and they are generally vulnerable to UV radiation and to creeping under stress; and “in situ” assembling of parts of the envelope would require a vast clean workshop (dust free environment);
- to get around the earth moving problem, which is a real « show-stopper » when the diameter exceeds, let us say, around ten meters, some propose to take advantage of geological natural formations (young craters, volcanic chasms), but this is not applicable to the present case, since it is not likely to find a series of such formations close enough to each other.
To cope with these obstacles, let us apply the same basic principles as for the habitats:
- exclude all large earth moving works, energy and working time consuming, that is all types of works where the volume of moved materials is proportional the diameter D to the power 3;
- in situ production of materials and products manufacturing; this is all the more critical than for the habitats since the surface area increases with the square of the diameter (D²) while the masse increases with the cube of the diameter (D3) A 30m dome would in theory be 27 times more massive than a 10m dome);
- exclude all large monobloc structures (such as single-piece inflatable envelopes) and favor structures made up of small size prefabricated modules, which do not need oversize workshops, hoisting and handling gear.
6.5 Hemispherical geodesic domes
The structure best incorporating these principles is the hemispherical geodesic dome. It would be made up of assembled triangular shaped window panes, made in tempered glass (same type of glass as used for the habitats), mounted in workshop on steel frames, via rabbets. These modules, each one some 2m wide and weighing around 300kg, would be easy to move on the building site and assembled, level per level, by a team of fitters using mobile positioning tools, driven by a robot. This robot should be autonomous and able to handle the panes, position them with a high level of precision, apply possible tightness coatings and proceed with relevant fastening (boltings). Such tasks are already performed by robots in the car industry. Operators would just supervize the job and cope with unexpected issues, therefore they would not need to put up with the stress resulting from sustained physical activity in spacesuits.
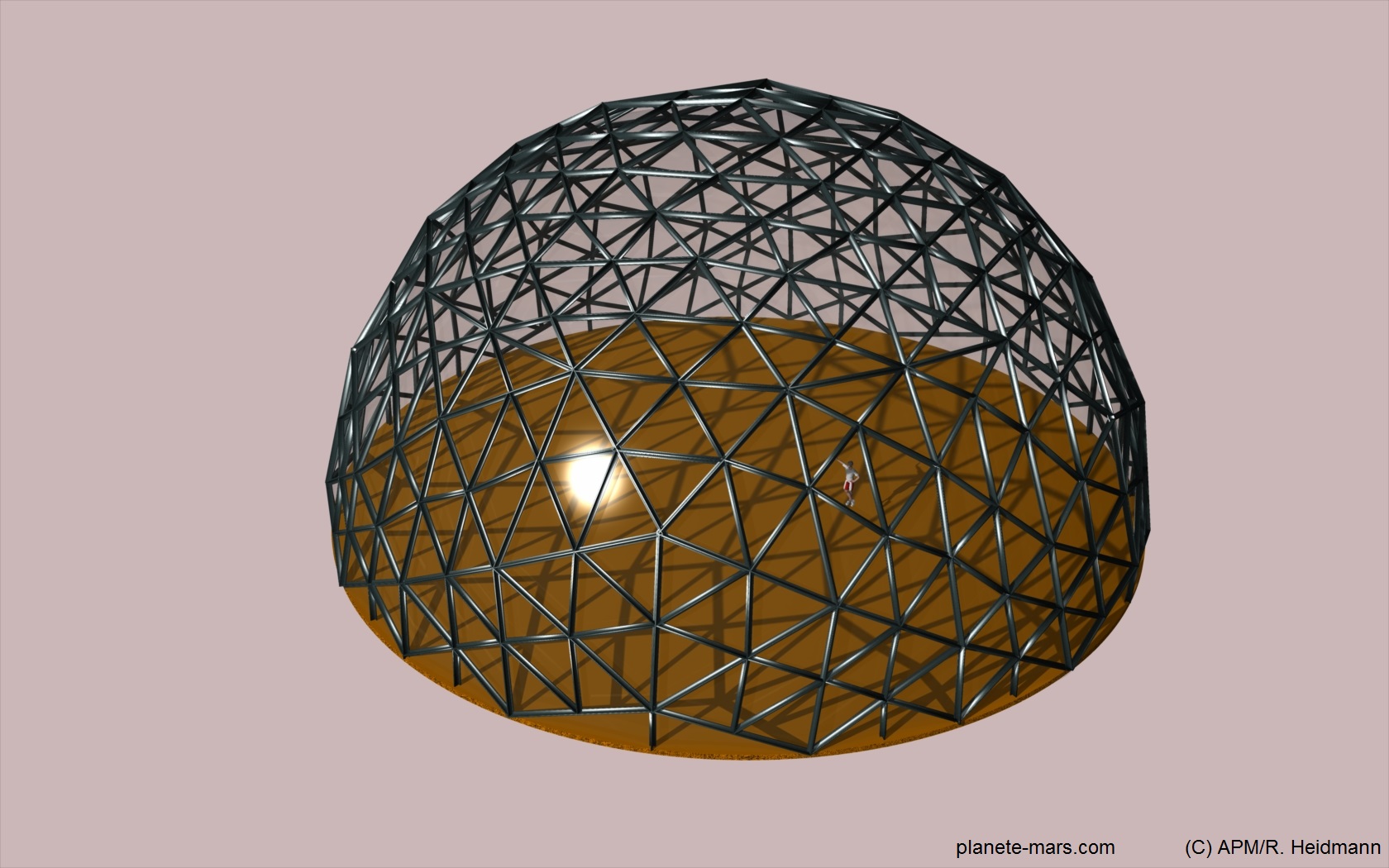
Geodesic dome, 30m diameter, flat glass panes and steel structure, erected on foundations in reinforced duricrete.
Choosing a hemispherical shape may avoid massive earth moving works, but leads to another problem: mooring the structure to the ground. Let us give some orders of magnitude for a 20m diameter dome (a modest size indeed) to understand the problem. The resulting vertical pressure force applied to the hemisphere reaches 15,700kN (around 1,600T); the circumference is 63m, so the mooring of the hemisphere must withstand a 25T/m effort, which means installing metal fittings able to transfer this effort to the ground. Actually, it will be necessary to erect this dome on a foundation sized to take in the strain, stiff enough to withstand the 1,600T force directly applied by the pressure, and with a level of distortion compatible with its physical integrity (also in the long run). This foundation should be made in duricrete, directly poured into a cylindrical form fitted with prepositioned interface metal fittings, and possibly reinforced by steel section bars. A one meter thickness should be enough (maybe a little more in the peripheral mooring area).
This is a major contruction work indeed, it will require a huge amount of duricrete, water and steel … But on the other hand, the quantity of materials required to build the dome itself will be divided by two and, above all, the earth moving works are significantly reduced.
Thinking about the possible layout of these areas led us to believe that 20m diameter domes would offer sufficiently wide areas to the community, provided of course that their number is proportional to the number of residents (two per “batch” of 100 residents).
6.6 Pleasant layouts, using the « cap » effect
We saw in section 4.1 that it was possible, for the condos as well as for the community areas, to design layouts featuring a) cleared areas with full lookout onto the outside (and subsequently full exposure to ionizing radiations), b) areas with partial visibility which look out onto a part of the Martian sky only, and c) completely protected areas, which will nevertheless be naturally lit by skylights.
In such a vast enclosure as allowed by a dome, the architects, while applying this layout principle, will give free rein to their imagination and come up with various kinds of configurations suited to the specific purpose of such or such place and provide the residents with a pleasant living environment, somewhat easing their feeling of being « shut off » from the outside. Let us say also that it is crucial to « fill up » the available volume of the dome as efficiently as possible, for if the settlers just made use of the ground area, the quantity of gas necessary to fill up the dome would be far excessive indeed compared to its benefit.
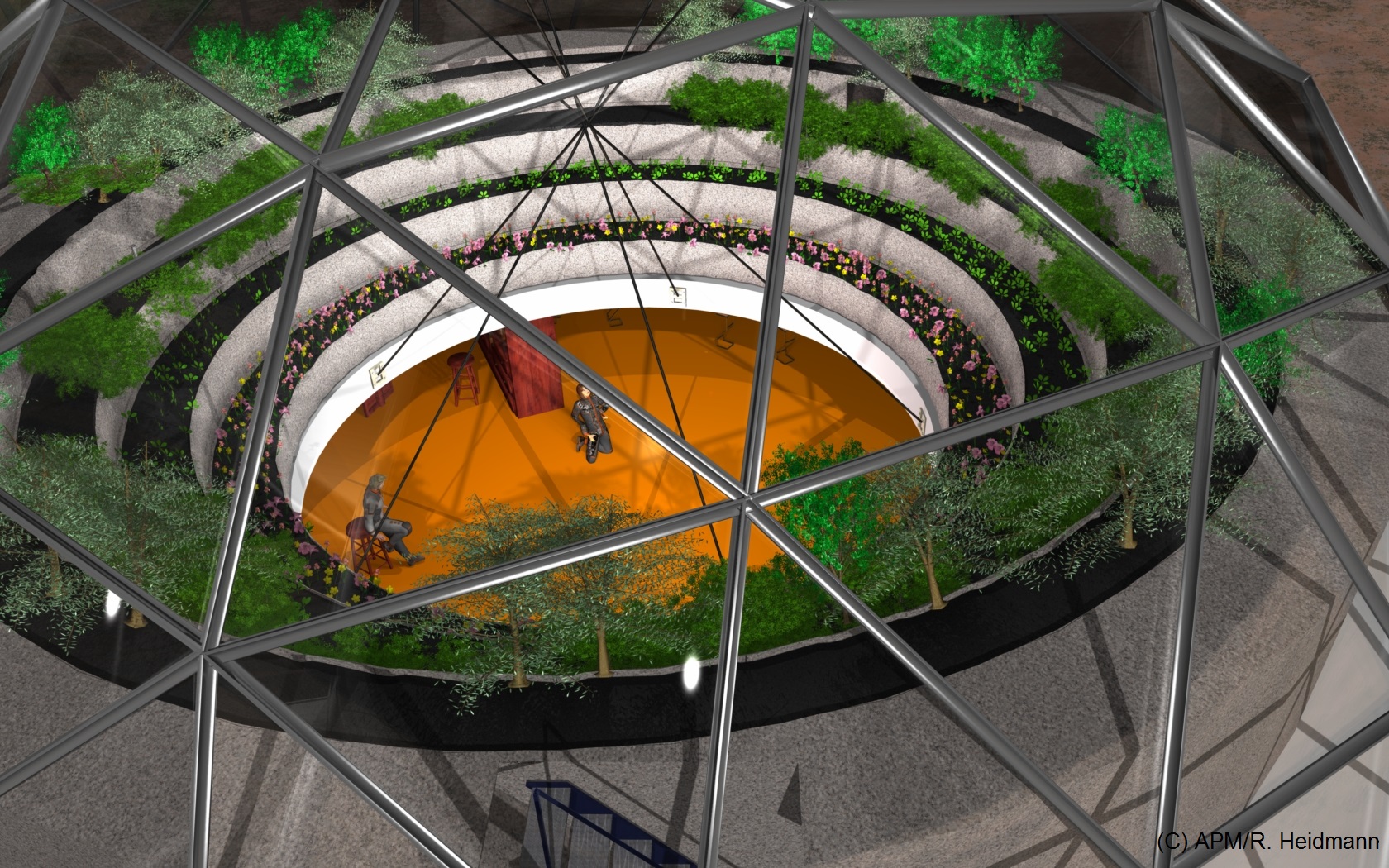
Top view of a 30m diameter restaurant-social area, such a large diameter is probably not realistic at an early stage of a settlement.
To better visualize this concept, find out possible problems arising from details and, also, to illustrate our point in order to see what it would actually look like, we designed two layouts, respectively a restaurant-social area and a fitness center (including a swimming pool!). In both cases, the « cap effect » is achieved by introducing a kind of circular amphitheater, fitted with hanging gardens, located above offices, laboratories and technical areas (completely protected). This structure rests on a scooped out disk, 20m outer diameter, 8m inner diameter and a ceiling height of around 2.3 m. Its weight is partially taken in by the dome structure, through tie-bars. Near the outside wall, each resident enjoys perfect protection from the radiation; in the center of the area, he is still partially protected by the slope of the terraces, but still he could decide to climb on top of the terraces to catch a panoramic view of the landscape or just for a moment of contemplation. Plantations bring the whole dome to life, while providing a lobby to those who enjoy gardening…
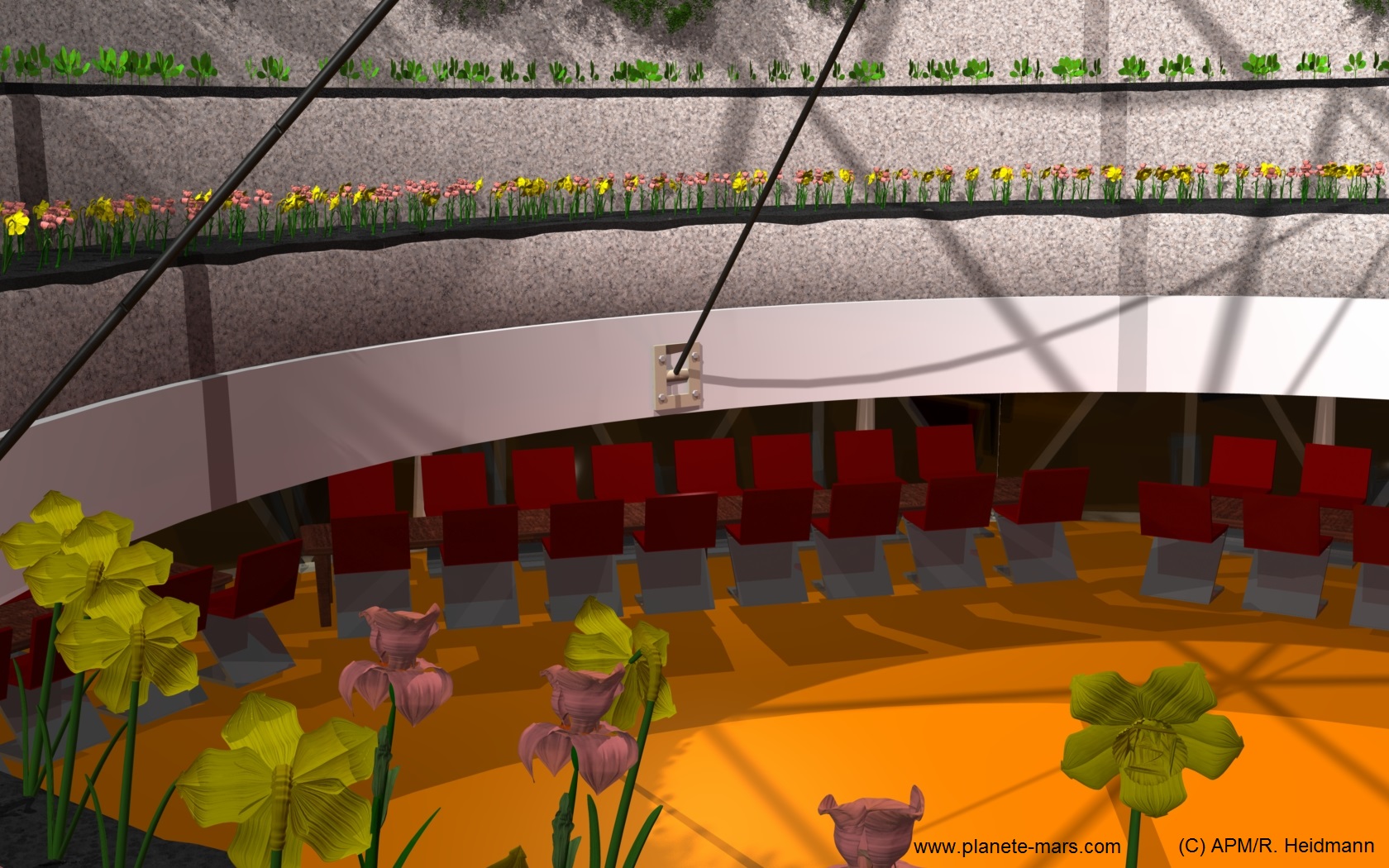
Restaurant area (one hundred seats in two shifts).
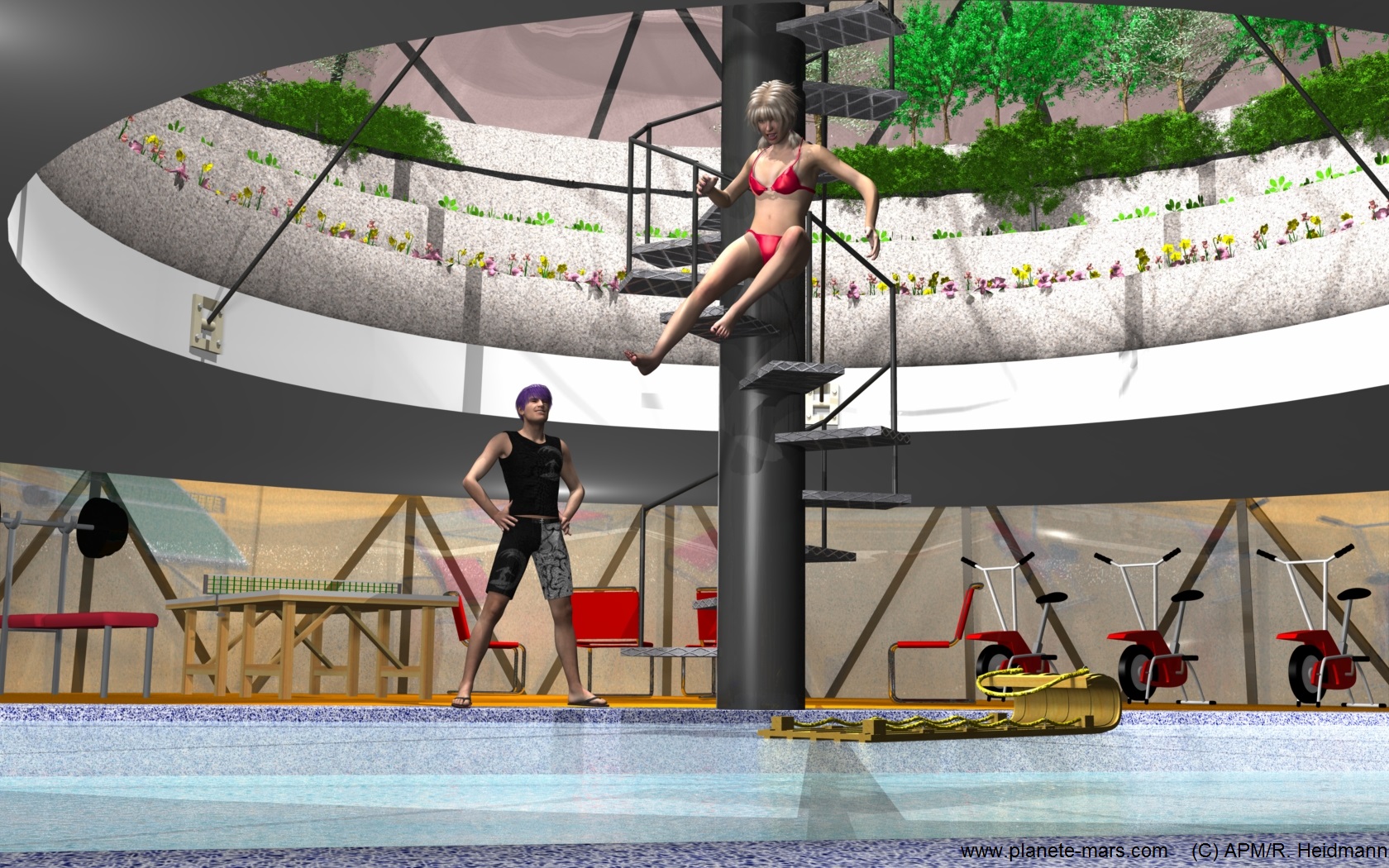
Diving in Martian gravity: a must for tourim activities.
6.7 Some sizing data
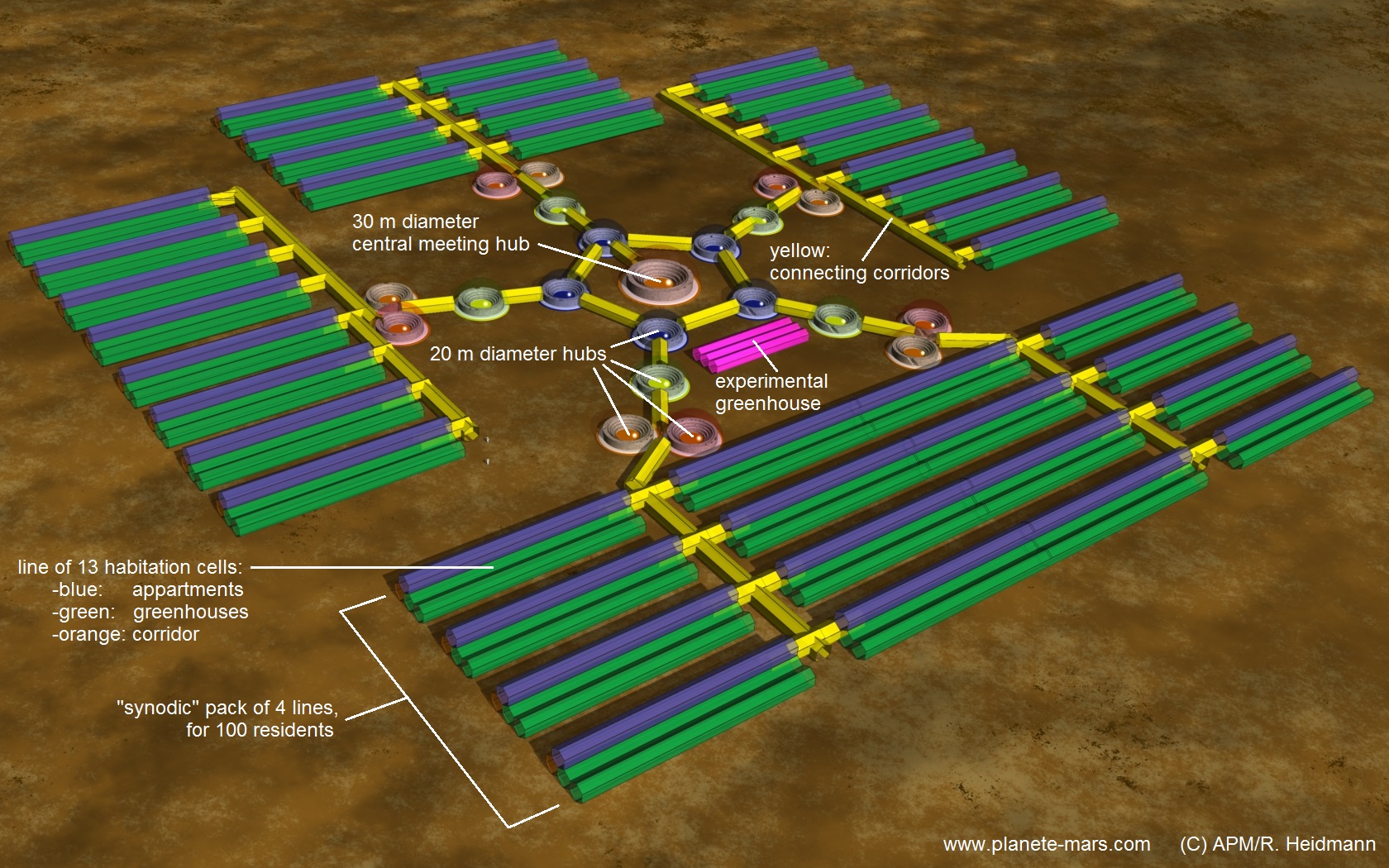
Possible arrangement of a settlement’s living areas (1000 residents).
7 Overview of production means for building purpose
7.1 Selecting materials sectors
We have listed in section §4.3 the various materials worth considering for habitats construction according to design principles detailed in section 4. Looking at the implementation of this construction process led us to select three main material sectors, both for habitats and community areas:
- the glass sector, for tempered glass flat windowpanes production (no forming required);
- the steel sector, for profiled angle bars production, designed for the frames of the windowpanes (steel is preferable to aluminum, which requires much more energy to produce);
- the duricrete sector, for domes foundations and relevant « terraces ».
Despite the great advantage of plastics’ relative lightness (compared to glass and steel), we do not recommend to develop a plastics sector, mainly because of its poor mechanical properties, low ageing resistance (UV radiation, creep) and flammability.
Also, brick laying has been considered only as an option (as well as “3D building”) to design the first community areas.
7.2 Glass sector
Producing glass may seem relatively easy, considering that the main raw material required, silica (SiO2), abounds on Mars, and that glass industry was already practiced more than two thousand years ago on Earth. In fact, even if a glass industry is not impossible in principle, its implementation on Mars requires importing significant production means from Earth.
The first step, harvesting enough raw materials, is not simple. Indeed, the basic process requires indeed silica (70%), but also soda (14%), lime (10%) and metallic oxides (magnesia, alumina, 5%). Harvesting silica is easy. But Martian silica is rich in iron oxyde, which must be eliminated; this can be done by a chemical reaction with carbon monoxyde (CO), byproduct of other chemical processes. Soda and lime are usually produced from carbonates, but carbonates are scarce on Mars, at least on the surface[8]. It can also be produced from calcite (5% content on Mars).
Metallic oxydes are easier to deal with. But this means that it will be necessary to: spot the rich areas (deposits), mine the materials, refine them, and possibly transform them in the open field, therefore we need mobile harvesting facilities and a kind of small mobile chemical processing unit.
The materials must be heated up to 1550°C and mixed in order to produce a homogeneous glass paste. This paste is then spread over a liquid[9] tinbath, its topside is burned by gas blowtorches (on Mars, this gas can be “in situ” produced methane); during the gradual cooling period occurring in this floating oven (from 1100°C down to 600°C) the glass sheet acquires perfect flatness on both sides. Finally this sheet is fed into a third oven, a reheating oven, where it is subjected to thermal treatments in order to acquire its mechanical properties. Once outside, the glass cools down, its sides are then cut off with diamonds to obtain calibrated blades which are finally cut out into windowpanes of the desired length and stored upright in transportation frames. This cutting process will be slightly more complex to produce the triangular panes of the geodesic domes.
[8] The Phoenix lander measured a 3 to 5% calcite concentration in Martian regolith.
[9] The « floating » process, discovered by the Pilkington company in 1959, revolutionized glass panes industrial production.
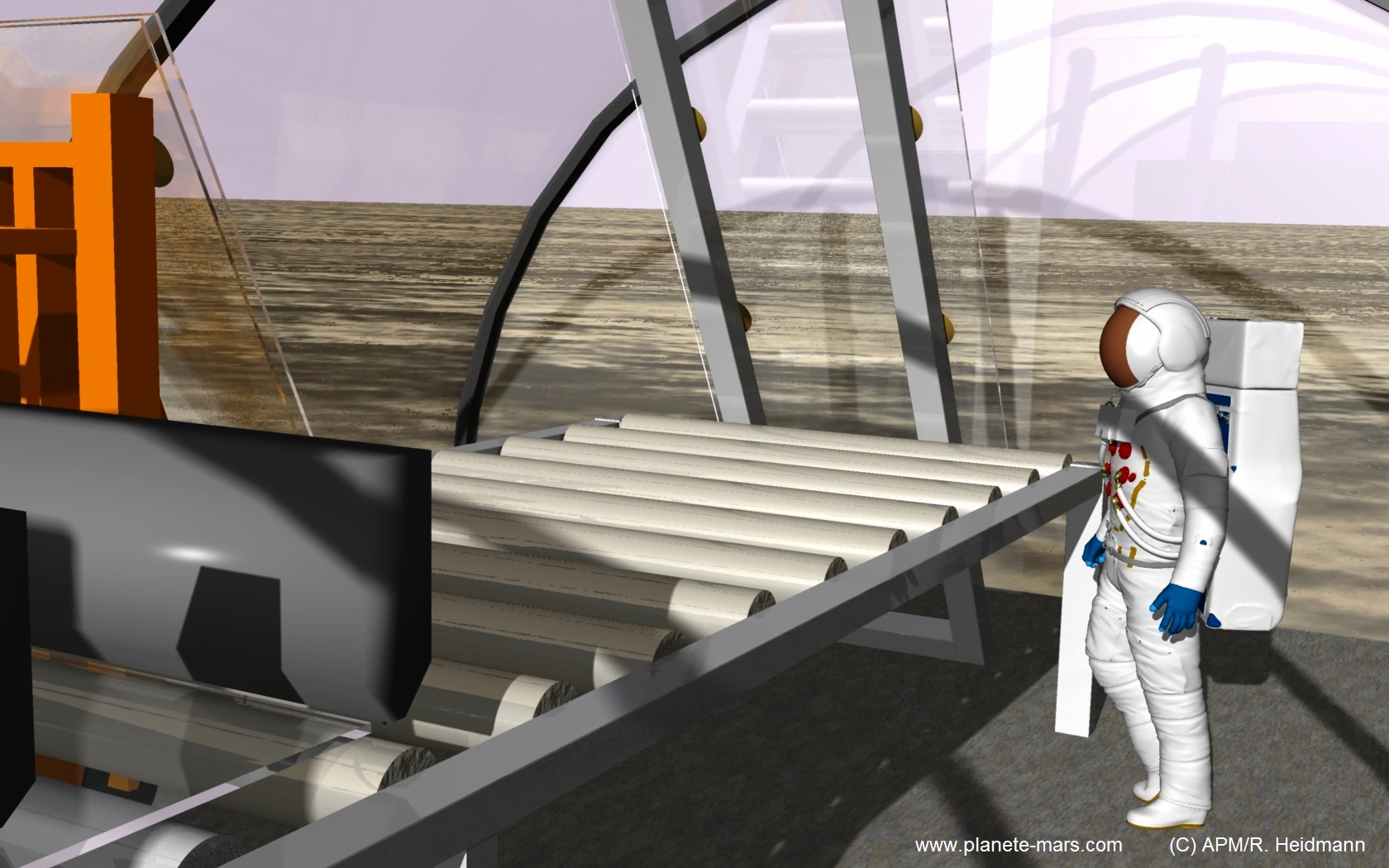
Cutting out and storing the glass panes, under a tent (not pressurized) for dust protection purpose.
The size of the processing chain depends on the desired width of the pane (1.5m in the present case) and on the desired production rate. In Earth industries, the reheating oven’s length may reach 150m; but here the production rate will be much lower, so we can afford a sheet moving at a much lower speed. Now, the various components of this chain will reach Mars ready for use, and their mass will be significant. On Earth, the ovens are supplied with natural gas; for the sake of efficiency we shall favour electric ovens whenever gas combustion is not required; but the floating oven requires a direct action of the heating gas upon the product; therefore it will be necessary to supply at least one part of the chain with LOX/CH4.
In order to drain the heat radiated by the ovens, these will be installed in the open air; the completion section only will be protected (from dust) by a plastic tent (not pressurized). Its operators will need to don spacesuits.
7.3 Steel sector
Implementing a steel based Martian metal working industry[10] implies a heavy facility and a lot of work; iron ores are available on Mars, but producing steel will require plants working at high temperature. Importing and installing such plants on Mars, and supplying them with energy and gas (CO, O2) will require a significant portion of the basic investment of a « small » settlement of 1000 residents.
The necessary raw material is available in huge quantities and easy to collect, this will be essentially hematite (Fe2O3). Then the oxide must be reduced to obtain iron; on Earth this is generally done in a blast furnace, which is fed with coke (almost pure carbon) and oxygen (air) and works at a 1200°C temperature. On Mars, carbon as such is not available; but we can easily obtain carbon monoxide, CO, as a byproduct of the thermal breakup of carbon dioxide of the Martian air which gives out oxygen (the other way to obtain oxygen is water electrolysis). Now CO happens to be the right chemical agent for iron reduction. This reaction is slightly exothermic, so a « blast furnace » can be operated with CO with a low amount of energy, provided we raise its temperature to 700°C or, even better, to 900°C.
In this way we shall obtain cast iron. To obtain steel, it is necessary to adjust the carbon concentration[11] to the required level, while « burning » impurities, then, if required, combine this metal with other metals (aluminium, chromium, manganese, silicon, titanium, vanadium…). But high strength steel or stainless steel is probably not necessary on Mars, since weights are three times lower than on Earth and Martian air is free from usual oxidizing agents on Earth (water, oxygen). For this purpose, on Earth, the cast iron leaving the « blast furnace » is brought into a refinement unit where it is blown with oxygen to burn out carbon in excess and residual impurities, up to 1200°C; then other metals can be added. On Mars, we could proceed with both operations successively in the same enclosure, by injecting first carbon monoxide, then oxygen, as far as necessary.
Provided its temperature reaches 1000 or 1200°C, steel can be extruded, which may be useful to produce the angle bars of the windowpanes. It would be beneficial to proceed with this shaping just after the refinement process, in order to avoid an additional cooling-heating cycle.
[10] Aluminum, the production of which out of alumina requires a lot of energy, remains indispensable for electrical wiring; a possible way to go would be recycling parts of the structure of landed vessels…
[11] Cast iron and steel differ by their carbon concentration: from 2.1% to 6.7% for cast iron and 0.03% to 2% for steel.
7.4 Duricrete sector
This ersatz of concrete can be produced right out of regolith and water, its production just requires a low temperature oven (electric oven). It may then be used as conventional concrete, either in precast, or in cofferings. Its mechanical characteristics, around 50% lower than concrete, can be improved by blending it with fibers (glass, Kevlar) which increase its resistance to cracking.
7.5 Needed quantities
Let us assume that for each synodical period, besides condos for 100 additional residents (50 batches), we need to build two community areas (including one restaurant-social area). Basing ourselves on estimates relevant to these areas and the 50 batches of habitats, we reach the following quantities to be produced during this period, to which we must add the materials necessary for roads and ancillary buildings (around 10% for glass and steel):
These quantities are reasonable; because the production is spread over a rather long period. On the other hand, we should realize that they will require a significant mining effort, assuming that the favorable harvesting areas have been spotted beforehand. Bear in mind that these quantities refer to living areas only, thus excluding energy production areas, resources processing and construction modules manufacturing areas. Also, production rates calculation does not account for maintenance and preparation time; therefore these values are minima.
The means enabling these production rates can be implemented on the Martian surface upon the first shuttle flight of the transportation fleet, the six vessels of the fleet will also bring a team of a few dozens pioneers in charge of this job, and the facilities necessary for their stay.
7.6 Energy needs
A large amount of heavy equipment will be necessary at an early stage of the settlement, the most significant of which will probably be the power plant supplying the energy required to produce the materials and parts at the rates mentioned above.
It is difficult to give an accurate estimate of the power needs, in particular the power necessary to heat up the enclosures operating at high temperature, and keep them running (indeed, their thermal balance depends on the walls’ thermal insulation), nevertheless we tried to estimate the power consumptions of the main manufacturing units. We also looked up at the existing literature, in particular the work of 4Frontiers (Homestead project).
Beware, this estimated value just covers the needs of the habitats construction; we must also consider the day to day energy needs of the settlement, which will grow linearly during the whole settlement period (around twenty years). By the way, this matter is discussed in our study « A Martian settlement, technical analysis », we assume in this study that the total electric power needs will average 30kWe per resident in steady state, which is 30MWe for a 1000 residents settlement. We assume also that the greenhouses will be lit naturally.
Taking into account the production rates, the “in situ” resources utilization process described on the figure of section 4.3, the physical properties of the materials and the power needs of the chemical reactions involved, we drew up the summary table below.
For each important process this table features the:
- Amounts of processed materials, in and out (tons per day);
- Required energy needs (kJ/kg), thermal or electrical energy;
- Corresponding powers (kW or MW).
This balance was set up considering that the energy is supplied by nuclear reactors (see next section) and that the heat of the cold source is put to good use too, but only for low temperature units (around 300°C), this will reduce the size of the heat exchanger and heat pipes necessary for heat transfers. This will apply to the following processes:
- Heating up living areas and greenhouses;
- Processing some ores and some parts of the materials processes (drying ovens, heating up Sabatier reactors …);
- Extracting water out of regolith through heating-distillation. However, for plants requiring much higher temperatures, this heating mode is impractical, even if the temperature of the cold source reaches 850°C. In this case, it is indispensable to use electric power (resistors or electric arcs) or provide heat through gas combustion (CH4 or CO + O2). This applies to:
- CO2 decomposition (1100°C);
- Windowpanes production (1550°C, then 1100 to 600°C);
- Steel production and heating for hot forming purpose (1200°C).
According to the Homestead study, the ratio between thermal and electrical energy consumptions is 8 to 3. Now, this study definitely favors bricklaying over glass; in the present case, the required amount of thermal energy would be much lower.
Noteworthy fact, water electrolysis clearly dominates the electrical energy needs, it requires a power of around 9MWe, and relevant water extraction facilities should produce some 10 tons of water per day. This quantity is considerable, but on the same order of magnitude as the needs of the residents and the greenhouses, which reach around 2.3 tons for 1000 residents (considering a 90% recycling rate). This is mainly due to the large amount of methane to be produced. Methane is a convenient fuel (together with liquid oxygen) for the various planetary vehicles (rovers)[12] used for mining various resources (their estimated methane consumption is circa 2.9T per day, which means twice this amount for a settlement of 2,000 residents as mentioned before, considering an average power of 1,100HP for the fleet of rovers). But to this quantity we must add the fuel to fill up the rockets for the return flight to Earth (this means an additional minimum amount of 1,320 tons of methane (6 rockets, 220 tons each); twice that amount in case of « immediate » return, for an opposition mission).
We see also that the direct thermal power is just one tenth of the total power, which means that it is not really relevant, considering the additional complexity involved.
To these power levels we should add also the power required for ancillary operations, such as compression and liquefaction (liquefaction of hydrogen is very costly) and account for various losses and unavoidable power leaks.
[12] As for planetary vehicles, methanol (CH3OH) is a more promising fuel than methane. It can easily remain in a liquid state, is denser, and can be produced in a Sabatier reactor, provided some modifications are brought to operational conditions.
7.7 Solar power: an attractive option, though unrealistic during the starting phase
Considering the relatively high level of electric power needed at a very early stage of the settlement, around 10MW, a power value which is bound to grow threefold during the building phase, solar power (photovoltaic) is definitely not an option. Indeed, in case of fixed solar panels, which are indeed the only reasonable option considering the high number of panels involved, taking into account all limiting factors (distance from the sun, seasons, height of the sun above the horizon, day-night cycle, dust storms) the average power should reach 60W/m2. Therefore, generating 10MW means installing right away panels covering a surface area of 170,000 m2, which corresponds to a 340m per 500m rectangle and to an impressive total of 34,000 panels, 5m2 each … The time spent to install and maintain these panels (in particular considering Martian dust) is a serious disadvantage. Anyway, the weight of such a solar power plant (panels, supports, electrical equipment, estimated total : 340 tons!), even taking into account an optimistic unit weight of 2kg/m2, makes it totally unrealistic to import it from Earth. Moreover, upon each synodical period an additional 110 tons should be imported and installed!
So, at least at an early stage of the settlement, nuclear power will prevail. But would it be reasonable to consider later in situ mass production of solar panels? This point of view is widespread, because silicon, which is an essential constituent of the solar panels, abounds in the Martian ground. Now, having a closer look at the processes required makes it an unrealistic option indeed, not so much due to the size of the facilities involved, but because of the high level of technical complexity of the industrial process. To summarize, we need to implement the following:
- From metallurgical silicon (silicon is obtained from quartz), producing a « solar » quality silicon; required purity: 99.9999%!
- Crystallizing this solar silicon to produce plates;
- Turning this crystallized silicon into photovoltaic cells;
- Assembling these cells into modules;
- Assembling these modules into panels, including all electrical and support accessories.
Silicon refinement process requires a lot of energy, and its doping (enrichment) is a very delicate task which should be carried out with an extreme care… all the while producing 65m2 panels area per day. This is not impossible, but should definitely be moved to a later stage of the settlement development, when the settlers will very likely benefit from significant progress of the photovoltaic technology.
7.8 Electronuclear generator
The Nuclear fission generator is already the preferred option for the first exploratory bases, temporary or permanent, whether on the Moon or on Mars. The main reason is that, for the power range required by the base, it represents the most compact, and easiest to install, power generating solution. Preliminary studies show that such a generator, sized for a permanent 40kWe output power, would weigh less than 5T, all inclusive. But such a nuclear core may operate at a higher power, without any significant increase of its size or fuel capacity, it may indeed release much higher thermal power to the cooling fluid, and within a higher temperature range[13].The factor limiting the output power of the generator is actually the size of the cold source – heatsink or heat exchanger[14].
If we choose the heatsink option, it is essential to raise its temperature (its radiated power capacity varies with the fourth power of the temperature, T4), same applies to the air input temperature of the turboalternator turbine. Increasing the temperature from 400 to 1,125K (850°C), with a turbine air input temperature averaging 1,400°C, multiplies the radiated power capacity by more than 60. For instance, doubling its surface, installing 4 panels crosswise, each segment 15m long, we reach 5MWe (order of magnitude). Therefore the settlement should be fitted with two such nuclear generators. Each generator would include two thermodynamical loops; thus providing for a two levels redundancy.
Obviously, increasing the heatsink input temperature up to 850°C makes its design and installation more difficult. Folding up the heatsinks during the transportation phase is not technically realistic, so it may be necessary to assemble the panels (and their fluid circuits) on site.
The nuclear generators’ lifespan, without any necessity to refuel, should reach 20 to 25 years, in order to get an operating time before revamp compatible with the time necessary to build up the settlement (assumed to be 20 years).
[13] The Nuclear experimental rocket engine NERVA, with a reactor diameter around one meter, managed to produce 4,000MWth in actual operation, the equivalent of more than 4 nuclear power plants!
[14] On Mars, unlike on Earth, no cold source such as rivers or seaside is available. Using the ground as a cold source would imply a large amount of earth moving activities. And the atmosphere is much too thin for this purpose (although some projects feature forced convection by motorfans).
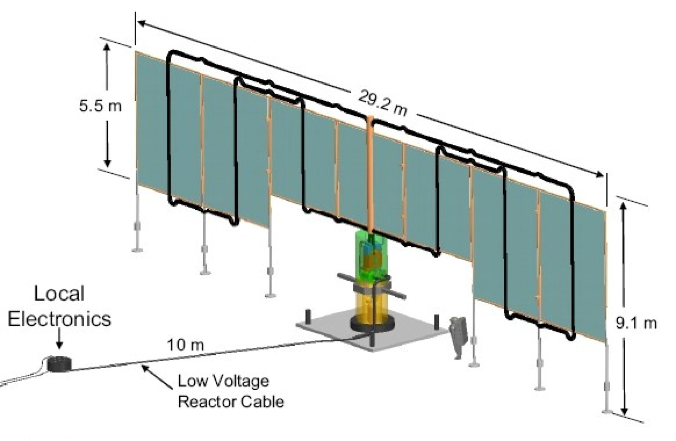
NASA project of a 40kWe planetary nuclear generator; the size of a possible 5MWe generator would be comparable, but it would feature 4 heatsink panels instead of 2. (doc. NASA)
Now, this technology has several drawbacks:
- it should be politically acceptable;
- even if the generators’ cores operate at low power, in the end they will run out of nuclear fuel and their materials will age; therefore it will be necessary to import new generators from Earth;
- it is also necessary to foresee the dismantling of the generators and, in the meantime, restrict the access to their installation area;
- in order to avoid long electric power lines and fluid pipes, the generators must be installed very close to the highest power consuming facilities (in the present case, the water electrolysis unit).
Despite these drawbacks, given the technology mastered so far, if solar power may be considered in the long run, the only reasonable option to power up a settlement of the size dicussed here (1000 residents) is definitely nuclear fission.
8 A technical analysis … but above all a human adventure
In the present study we have proposed a draft design of the habitats of a Martian settlement. The choice of this design essentially results from the chosen settlement scenario, a scenario favouring a settlement of 1,000 residents on Mars (either tourists or scientists). An economic model, still under development, proves that such a scenario is realistic, provided some financial constraints are accounted for, mainly regarding travel cost and the amount of the initial investment.
Its design followed a few guidelines, the most important of which are:
- on the one hand, resorting as far as possible to local resources, all the while knowing that the transportation mode makes it possible to organize a significant cargo traffic from Earth (300 tons per synodical period);
- on the other hand, since we are dealing with a residence, also designed for adventure tourism, potential customers should be offered the most attractive living conditions possible, in particular we should definitely refrain from burying them in “molehills”.
It was necessary to study the consequences of these main principles for the design and construction of these habitats in order to secure the feasibility of this type of settlement and to obtain the costing data used to prepare the economic model.
On the technical side, the analysis does not highlight any fundamental obstacle. However, it shows that the industrial facilities which must be imported from Earth are considerable and that resorting to nuclear fission is compulsory. Water is an essential ingredient too, for it is an indispensable resource to produce the energy vector needed (propellant) by the vehicles, whether interplanetary rockets or large rovers operating in the mining areas and habitats production areas.
Behind this huge technical infrastructure, there will be human beings. It will be essential to care for the satisfaction and well being of these residents, paying customers and providers of paid services, and keep them in good physical and mental condition. But also, and first of all, a small team of pioneers will need to carry out successfully, in a much more austere living environment and under the relentless pressure of the synodical cycle, a complex and innovative series of tasks which will benefit from the considerable funds invested by an association of bold private entrepreneurs and space agencies.
Conclusion
The concept described above, based on flat glass technology, is not conventional, but it does meet the requirements of a « residential base » scenario. Now, manufacturing the windowpanes presents real technical challenges, whether provisioning additives or implementing the production ovens. Mounting the windowpanes in steel structures will definitely not be easy either. This study therefore should be regarded more as a preliminary and exploratory research rather than a recommended solution.
It is essentially characterized by the adoption of a « business » scenario (offer of a Martian residence). This point of view has not really been considered yet, indeed the few « business case» studies done so far are mostly based on the principle of export of goods, whether material or immaterial, it is not easy to put actual figures behind such an ill defined concept. In fact, most of the actors, mainly institutions, consider the settlement as a natural extension of scientific exploration. Now, without any commercial perspective, the incentive for such a huge investment simply is not there. And yet, on top of that we should assume that some extremely rich visionary entrepreneurs will show some inclination to philanthropy, considering the risk and the long time for the return on investment.
I guess we can see now the first signs of this new era, which will represent for the space agencies an opportunity to revive the development of their space activities.
Richard Heidmann, Association Planète Mars vice president – English translation: Etienne Martinache.
References
Books and communication collections
Embarquement pour Mars, 25 défis à relever – Collectif d’auteurs Association Planète Mars, 2015 (2e édition), A2CMédias (Eyrolles)
Cap sur Mars (trad. française de « The Case for Mars ») – Robert Zubrin & R. Wagner, Editions Goursau ISBN 2-904105-09-3
Mars, Prospective Energy and Material Resources – Viorel Badescu, Springer, 2009, ISBN 978-3-642-03628-6
From Imagination to Reality, Part II : Base Building, Colonization and Terraformation – American Astronautical Society (AAS) Vol.92, 1997 – ISBN 0-87703-428-X
Strategies for Mars: a Guide to Human Exploration – AAS Vol.86, 1996 ISBN 0-87703-406-0
The Case for Mars V – AAS Vol.97, 2000 ISBN 0-87703-460-5
Resources of Near-Earth Space – The University of Arizona Press, 1993 ISBN 0-8165-1404-6
Planète Mars, une attraction irrésistible – R. Heidmann, ALVIK Editions, 2005 ISBN 02-914833-36-9
Articles (other than those found in AAS collections above)
Analyse d’un concept « Mars Colonization Transport » (MCT) a deux lancements – R. Heidmann, Sept.2015, site planete-mars.com
Une colonie martienne : essai d’analyse technique – R. Heidmann, Nov.2008, site planete-mars.com
Resources Utilization and Site Selection for a Self-Sufficient Martian Outpost – G. James, G. Chamitoff, D. Baker, 1998 NASA/TM-98-206538
In Situ Resource-Based Lunar and Martian Habitat Structures Development at NASA/MSFC – M. P. Bodifor et al. , 2005 AIAA 2005-2704
Requirements for Space Settlement Design – A. E.Gale, R. P.Edwards, The Boeing Company 4Frontiers Corporation
A Permanent Settlement on Mars: Generation 1 Design – Georgi Petrov, B. Mackenzie, M. Homnick,. Palaia, IV 4Frontiers Corporation
Martian Cement – Robert J.Milligan, 2007 10th Mars Society Convention
MERIT: New Approach for a Large Scale Space Infrastructure based on Resources from Mars – J. Powell, G. Maise, J. Paniagua STAIF 2005
Reference Reactor Module for the Affordable Fission Surface Power System – D. I.Poston, R. J.Kapernick STAIF 2008
A Greenhouse for Mars and Beyond – Christopher P. Rahaim, Paul A. Czysz STAIF 2008
Utilizing Martian Resources for Life Support – C. MCKay
A View of Future Human Colonies on Mars – Robert J. Gustafson, 2003, Orbital Technologies Corp.
Plexiglas – Optical & Transmission Characteristics – Arkema, 2000
Transparent Spinel Ceramics – TA&T Inc.
The Surface Endoskeletal Inflatable Module – C. M. Adams, Futron, NASA JSC
Éléments de technologie verrière – N. El Jouhari, universite Mohammed v–Agdal
Systèmes photovoltaïques : fabrication et impact environnemental – HESPUL, 2009
The Mars surface environment and solar array performance – Paul M. Stella and Jennifer A. Herman, Jet Propulsion Laboratory
Assessment of architectural options for surface power generation… – Chase Cooper et al., Acta Astronautica, 2010 Massachussets Institute of Technology
Web
Mars Foundation documents
4Frontiers documents
The Mars Society
Association Planète Mars (French section of the Mars Society)